Research
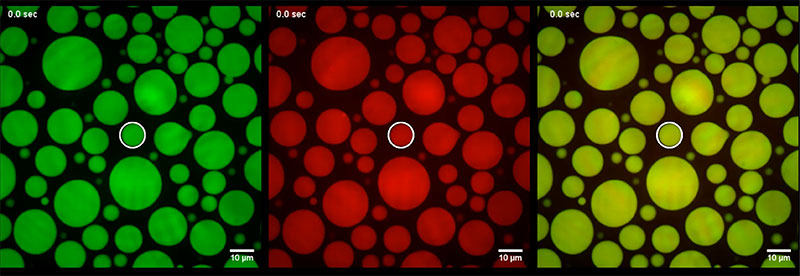
Djinovic-Carugo et al. Science Advances, 7, 2021
While the identification of LLPS-related phenomena in different areas of molecular biology research keeps growing at a rapid pace, our understanding of the underlying microscopic, atomistic-level details lags significantly behind. Namely, the state-of-the-art in the LLPS field is currently dominated by detailed cell-biological studies identifying the presence of LLPS condensates and establishing potential links with their biological function, biophysical studies assessing the mechanical as well as physicochemical properties and composition of condensates and, finally, largely phenomenological, mean-field physical theories explaining the general features of their phase diagrams. In addition, progress has also been made in understanding the determinants of LLPS when it comes to coarse-grained features of the biomolecules involved.
On the other hand, the question of the high-resolution structure and dynamics of LLPS condensates and their link with biological function remains a major challenge. What is the architecture of these intermolecular networks (meshes) at the atomistic level? How dynamic are they microscopically? In what way are they affected by post-translational modifications? How do microscopic features of LLPS condensates relate to their meso- and macroscopic characteristics and, most importantly, their function? In light of this, what are the physical and functional differences between biomolecular clusters and LLPS condensates? Many of these and similar questions remain to be answered. Finally, biological information is primarily encoded in the primary sequences of biomolecules and, in particular, proteins and nucleic acids. While the coarse-grained grammar of how sequence information translates to the level of condensates has been outlined in the case of some proteins, this is still largely missing when it comes to nucleic acids. The latter, however, are frequently an important part of LLPS domains and can phase-separate on their own. In both cases, moreover, the atomistic picture of how sequence information affects condensate behavior is still significantly incomplete. In short, there is an urgency to provide a fundamentally microscopic, quantitative picture behind different LLPS phenomena, leveraging the power of high-resolution experimental techniques and cutting-edge theoretical and computational approaches.
The principal aim of ELLIPSE is to address this challenge through a systematic, hypothesis-driven research in several, judiciously chosen test systems. The two key questions that are addressed are:
1. what is the relationship between the atomistic structure, dynamics, interactions and modifications of individual biomolecules undergoing phase separation and the macroscopic properties of the resulting LLPS domains?
2. which microscopic features and mechanisms link the structure and dynamics of LLPS domains with their biological, physiological function?
ELLIPSE addresses these questions by creating a multidisciplinary, highly-collaborative, three-pronged research structure, which will furthermore be reflected in program’s educational philosophy. The corners of this structure are defined by three different classes of methods represented by the participating faculty i.e. theoretical and computational approaches of biophysical chemistry and soft-matter physics, high-resolution techniques of structural biology, and biochemical and cell-biological functional measurements. The key element of this framework is a set of carefully designed PhD projects, each of which combines the whole spectrum of theoretical and experimental approaches as described above and addresses different questions in the area of LLPS in an integrative, multifaceted manner.